The three dimensional interactions of liquid water is difficult to study due to it’s fluid state but much has been learned by examining the structure of the ice crystals.
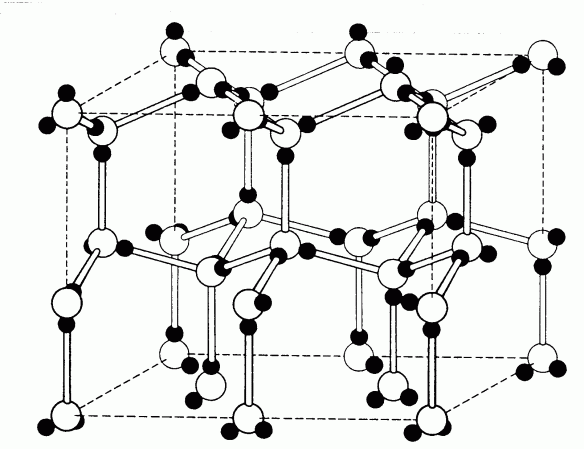
Ice Crystal Lattice. Click on image for credit.
Four adjacent hydrogen-bonded oxygen atoms occupy the vertices of a tetrahedron (tetra = four, hedron = plane, thus four-sided figure). The average energy required to break each hydrogen bond in ice has been estimated to be 23 kJ mol-1.
The ability of water molecules in ice to form four hydrogen bonds and the strength of these hydrogen bonds give ice an unusually high melting point. This is because a large amount of energy, in the form of heat, is required to disrupt the hydrogen-bonded lattice of ice. When ice melts, most of the hydrogen bonds are retained by liquid water, but the bonds are distorted relative to those in ice, so that the structure of liquid water is more irregular.
Interesting point to note is that the density of most substances increase on freezing as molecular motions slows and tightly-packed crystals form. The density of water also increases as it cools until it reaches a maximum at 4°C (277K). Then, as the temperature drops below 4°C, water expands. This expansion is caused by formation of the more open hydrogen-bonded ice crystal in which each water molecule is hydrogen-bonded rigidly to four others. As a result, ice, with its open lattice, is less dense than liquid water, whose molecules can move enough to become more closely packed. Because ice is less dense than liquid water, ice floats and water freezes from the top down. This has important biological implications since a layer of ice on a pond insulates the living creatures below from extreme cold.
Furthermore, two additional properties of water are related to its hydrogen-bonded characteristics- its specific heat and its heat of vaporization.
The specific heat of substance is the amount of heat needed to raise the temperature of 1 gram of the substance by 1°C. A relatively large amount of heat is required to raise the temperature of water because each water molecule participates in multiple hydrogen bonds that must be broken in order for the kinetic energy of water molecules to increase. Btw, the abundance of water in the cells and tissues of all large multicellular organisms means that temperature fluctuations within cells are minimized. This feature is of critical biological importance since the rates of most biochemical reactions are sensitive to temperature.
The heat of vaporization of water is also much higher than that of many other liquids. A large amount of heat is required to convert water from liquid to gas because hydrogen bonds must be broken to permits water molecules to dissociate from one another and enter the gas phase. Because the evaporation of water absorbs so much heat, perspiration is an effective mechanism for decreasing body temperature; the sweat will absorb heat away from the body as it evaporates.
As discussed earlier, water molecules are permanent dipoles that are polar and can interact with and dissolve other polar compounds and compounds that ionize (the latter are called electrolytes). They can align themselves around the ions formed from electrolytes so that the negative oxygen atoms of the water molecules are oriented toward the cations (the positive ions) of the electrolytes and the positive hydrogen atoms are oriented towards the anions (the negative ions). Consider what happens when a crystal of table salt- NaCl (sodium chloride) dissolves in water. The polar water molecules are attracted to the charged ions in the crystal resulting in sodium and chloride ions on the surface of the crystal dissociating from one another, and the crystal beginning to dissolve.

Sodium Chloride dissociation (hydration) in water. Click on image for credit.
Each dissolved Na+ attracts the negative ends of several water molecules, whereas each dissolved Cl– attracts the positive ends of several water molecules (see diagram).
The shell of water molecules that surrounds each ion is called a salvation sphere and usually contains several layers of solvent molecules. A molecule or ion surrounded by solvent molecules is said to be solvated. When the solvent is water, such molecules or ions are said to be hydrated.
Thus, any polar molecule has a tendency to become solvated by water molecules. Ionic organic compounds, such as carboxylates and protonated amines, owe their solubility to the amino, hydroxyl, and carbonyl groups. Molecules containing such groups disperse among water molecules, with their polar groups forming hydrogen bonds with water.
Of course, the number of polar groups in a molecule affects its solubility in water. Solubility also depends on the reaction of polar to nonpolar groups in molecule: for example, one-, two-, and three-carbon alcohols are miscible with water, but larger hydrocarbons with single hydroxyl groups are much less soluble in water.
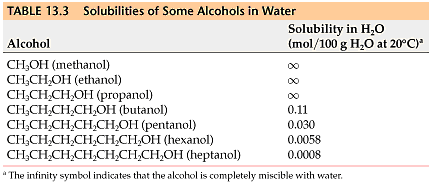
Table measuring solubility of molecule in water as it's non-polar hydrocarbon chain grows. Click on image for credit.
In a large molecule, the properties of the nonpolar hydrocarbon portion of the molecule override those of the polar alcohol group and limit solubility.